As the climate crisis accelerates, strategies to reduce atmospheric carbon dioxide (CO₂) are urgently needed. While technologies like carbon capture and storage (CCS) gain attention, an often overlooked but powerful ally lies beneath our feet: healthy, carbon-rich soil.
Soil carbon refers to carbon present in organic and inorganic forms in the soil. The majority exists as soil organic carbon (SOC), derived from plant residues, microbes, and other organic materials. Inorganic carbon includes carbonates, especially in arid regions, but plays a smaller role in active carbon cycling.
Organic carbon in soil is a key indicator of soil health. It improves soil structure, water retention, nutrient cycling, and biodiversity. But its most crucial role today is its ability to store atmospheric carbon dioxide.
How Soil Stores Carbon: The Science
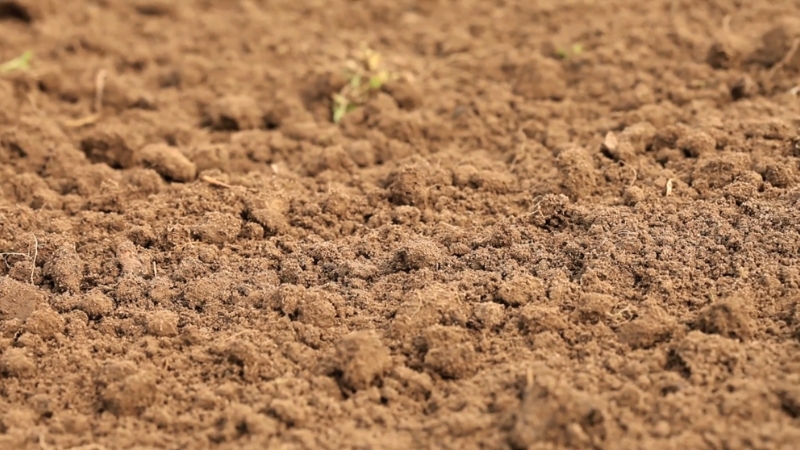
Plants capture CO₂ through photosynthesis and convert it into sugars and other organic compounds.
Some of this carbon is used to grow roots and leaves; some is exuded into the soil through root exudates—sugars and amino acids released to feed beneficial microbes.
When plant material dies or sheds, it becomes litter (leaves, stems, roots) that decomposes and contributes carbon to the soil.
Microbial Processing
Soil microbes—bacteria, fungi, and archaea—play a critical role. They break down organic matter, incorporating some carbon into their own biomass and transforming the rest into:
- Labile carbon: Easily decomposable, short-term storage
- Stable (humified) carbon: Resistant to decomposition, long-term storage
- Mineral-associated organic matter (MAOM): Strongly bonded with soil particles, highly stable
Physical Protection
Carbon in soil becomes stable when it is:
- Chemically bound to minerals
- Physically protected inside soil aggregates
- Stored in deep soil layers
These mechanisms can trap carbon for decades to centuries.
Types of Soil Carbon
Soil carbon isn’t a single substance—it exists in multiple forms that behave differently in the environment. Particulate organic matter, for instance, is derived from decomposing leaves, stems, and roots. It is vital for short-term nutrient cycling and microbial activity, but it can break down quickly if soils are tilled or left bare. Microbial biomass carbon, although short-lived, is crucial as an intermediary in carbon cycling, feeding other microbes and converting plant matter into more stable forms. The most valuable form in terms of long-term storage is humus, a stable form of soil organic carbon that forms through the microbial transformation of organic residues. Humus binds to minerals and gets stored in soil aggregates, reducing its exposure to oxygen and microbial attack—this is the key to locking carbon away for centuries. Inorganic carbon, while more chemically stable, is mostly found in arid regions and is less dynamic in the context of active carbon sequestration strategies. Globally, soils have immense potential to store more carbon than they currently do. The top meter of soil worldwide holds approximately 1,500 gigatons (Gt) of organic carbon—about three times the amount of carbon in the atmosphere. If managed wisely, even small percentage increases in soil organic carbon (SOC) can have a global impact. Let’s put this into perspective with real-world scenarios: This international initiative suggests that increasing global soil organic carbon stocks by 0.4% annually (or 4 per 1000) would compensate for the yearly increase in global CO₂ emissions. Though ambitious, it’s based on solid science. Soil’s carbon storage potential varies by region: For example, degraded soils in India and Africa show rapid gains when restored, while northern peatlands already store high levels of organic carbon and must be protected from drainage or burning. These examples demonstrate that soil is not just a passive recipient of carbon, but an active component in carbon cycling. Through informed land management, this carbon sink can grow—and help us buy time in the fight against climate change. Transitioning to carbon-smart agriculture isn’t theoretical—it’s happening across the world with measurable results. Here are six key practices that have been scientifically shown to increase soil carbon levels and improve land resilience. Conventional plowing exposes soil to oxygen, accelerating the breakdown of organic matter and releasing CO₂. No-till farming limits soil disturbance, helping preserve soil structure, moisture, and microbial life. Studies show that no-till soils can store significantly more carbon over time, particularly when combined with cover cropping and diverse rotations. Cover crops like clover, rye, or vetch are planted between main cash crops to keep the soil covered and biologically active year-round. They suppress weeds, reduce erosion, and increase biomass inputs into the soil, boosting microbial activity and organic matter formation. 🚜IIASA researchers explored the potential of carbon sequestration on farmland to combat #ClimateChange, finding that by 2050, these practices may cut emissions as effectively as planting new forests, while also increasing productivity and resilience. — IIASA (@IIASAVienna) September 23, 2024 Agroforestry integrates trees with crops or livestock systems. Trees store carbon both above ground (in trunks and leaves) and below ground (in extensive root systems). Their leaf litter adds organic matter to the soil, and their roots help build soil structure and reduce erosion. Applying composted organic matter—whether from food waste, animal manure, or green waste—directly feeds soil microbes and increases the formation of stable soil carbon compounds. This is especially effective in degraded or nutrient-poor soils. Biochar is a carbon-rich substance produced by heating organic material in low-oxygen environments (pyrolysis). When added to soil, it resists decomposition and can remain stable for hundreds or even thousands of years. Biochar also enhances water retention and provides a habitat for beneficial microbes. Well-managed grazing mimics the natural movement of wild herbivores, allowing grasses to rest and recover. This enhances root growth, promotes carbon inputs into the soil, and increases ground cover. Despite its potential, soil carbon sequestration isn’t a silver bullet. There are real-world limitations to what it can achieve, and understanding them is crucial for setting realistic expectations and policies. Carbon stored in soil can be quickly released if the land is mismanaged. Tilling, overgrazing, or clearing forests can undo years of sequestration efforts in a single season. Soils don’t have infinite storage capacity. As carbon levels rise, the rate of additional carbon storage slows and eventually plateaus. This means early gains are more significant, but long-term sequestration requires continuous effort and innovation. Accurately measuring changes in soil carbon is complex and expensive. Carbon stocks vary by soil depth, location, moisture, and microbial activity, making it difficult to create universally applicable models. Emerging technologies like remote sensing and machine learning may help overcome this in the future. Rising temperatures can increase microbial respiration in soils, leading to faster decomposition and CO₂ release. Warming may reduce the effectiveness of soil carbon sequestration in some regions, especially permafrost zones. Scientific research from around the world continues to validate the carbon-storing power of soil-based practices. The Rodale Institute’s 40-year Farming Systems Trial compared conventional vs. organic systems. Results showed: Source: Rodale Institute, 2020. A comprehensive global review found: Source: Lal, R. et al. (2020). Soil & Tillage Research In tropical soils, biochar amendments: Source: Lehmann, J., et al. (2003). Nature and Soil Biology & Biochemistry Soil is a natural climate ally with untapped potential. By managing land with carbon in mind, we can draw CO₂ from the atmosphere and store it safely underground, often for centuries. Unlike high-tech solutions, this approach is low-cost, readily available, and comes with added benefits: While not a substitute for reducing fossil fuel emissions, soil carbon sequestration can play a crucial role in a multi-pronged climate strategy, especially when supported by policy, investment, and farmer training. The soil beneath our feet holds part of the answer to one of humanity’s biggest challenges. It’s time we started treating it that way.
Type
Description
Longevity
Particulate Organic Matter
Fresh plant residues and litter
Months–Years
Microbial Biomass Carbon
Living microbial content
Days–Weeks
Humus / Stable Organic Carbon
Transformed and stabilized organic matter
Decades–Centuries
Inorganic Carbon
Carbonates, mostly in dry soils
Centuries
How Much Carbon Can Soil Store?
Carbon Pool
Estimated Storage (Gt C)
Atmosphere
~830 Gt
Vegetation (biomass)
~560 Gt
Soil (0–1m depth)
~1,500 Gt
Soil (0–2m depth)
~2,400 Gt
What Do These Numbers Mean Practically?
Case Example: French “4 per 1000” Initiative
Soil Type and Regional Variability
Region
Carbon Storage Potential (t C/ha/year)
Soil Type
Temperate Grasslands
0.3–1.0
Mollisols, Alfisols
Tropical Forest Soils
0.2–0.6
Oxisols, Ultisols
Arid Soils
0.05–0.3
Aridisols
Boreal Forest Soils
0.1–0.4
Spodosols, Histosols
Practices That Enhance Soil Carbon Storage
1. Conservation Tillage or No-Till Farming
2. Cover Cropping
3. Agroforestry
🔎 https://t.co/pTlML6uFqP… pic.twitter.com/Z2mo3xkDFn4. Compost and Manure Application
5. Biochar Application
6. Rotational Grazing and Pasture Management
Challenges and Limitations
1. Reversibility
2. Saturation
3. Measurement and Verification
4. Climate Sensitivity
Research and Case Studies
Case Study: The Rodale Institute (Pennsylvania, USA)
Meta-Analysis: Lal et al. (2020)
Biochar Study: Lehmann et al. (2003)
Conclusion